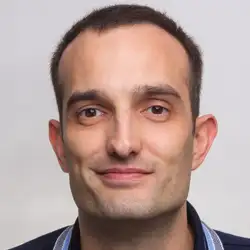
Written by Dr Matthew Roberts
Lead Consultant
Unlocking the mysteries of imaging radar data processing: an exploration
This article draws on the comprehensive guide “A Programmer’s Introduction to Processing Imaging Radar Data”, written by Matthew Roberts, Lead Consultant for Plextek.
The intricate dance of electromagnetic waves and their interaction with the environment forms the basis of radar technology, an essential tool in today’s technological arsenal. Radar systems, through their ability to sense distances and object characteristics, have found applications across numerous fields, from meteorology and automotive safety to military surveillance and air traffic control. Among the various types of radar systems, imaging radar stands out for its ability to not just detect objects but to provide some detail for the overall scene. For example, imaging radars allow you to ‘see’ the outline of a building, or even the layout of an office given a suitable configuration, providing invaluable insights into the observed scenes.
Introduction to imaging radar technology
Imaging radar operates by emitting electromagnetic waves and capturing their reflections from objects within its field-of-view. Unlike conventional radar that provides specific information on detected objects such as distance, speed, and bearing, an imaging radar coherently processes the received signals to construct a two- or three-dimensional image of the target scene. This capability makes it immensely valuable for applications requiring detailed spatial awareness and object identification, such as satellite earth observation, autonomous vehicle navigation, and landscape mapping.
One significant advancement in imaging radar technology is the Frequency Modulated Continuous-Wave (FMCW) radar. FMCW radar offers advantages over pulsed-Doppler ranging techniques, particularly for short-range systems, where wideband systems can be realised to achieve fine resolution at millimetre-wave frequencies, making it well-suited for mobile applications and devices requiring continuous monitoring of surroundings. As a leading innovator in the field of electronics and communication technology, we developed a millimetre-wave radar demonstrator. This FMCW radar operates in the 60 GHz license-exempt band with state-of-the-art in electronically scanned radar systems.
The components of an imaging radar system
The heart of our imaging radar system lies in its clever architectural design, comprising four main components: a computer, a digitiser, a radio frequency (RF) front-end, and a waveguide horn antenna. The RF front-end is responsible for generating the millimetre-wave signals transmitted by the antenna and for demodulating the received signals. The digitiser, in turn, converts the analogue signals into their digital counterparts, making them suitable for computer processing. The antenna steers its main lobe as the frequency of the transmitted waveform changes. This setup allows the radar to swiftly scan its field-of-view without mechanical movement, relying instead on electronic scanning facilitated by changing the frequency of the transmitted waveforms.
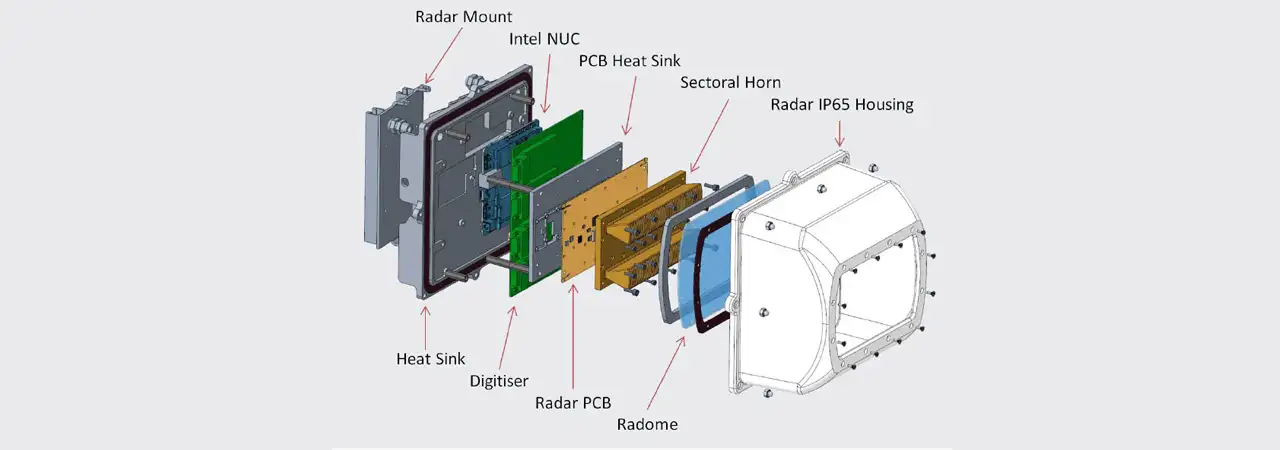
Imaging radar: RRI and raw data
In the world of imaging radar, the Ramp Repetition Interval (RRI) (equivalent to the pulse repetition frequency in pulsed radar systems) is a critical concept. The RRI refers to the period during which the FMCW radar’s transmit frequency is modulated. In FMCW ranging techniques, this is typically done by transmitting a linear chirp that incrementally rises at a prescribed rate (up-ramp) before being quickly reset (down-ramp) to its starting frequency. This modulation pattern is pivotal for defining the radar parameters such as range and Doppler resolution, frame rate, instrumented range, and mitigating against aliasing effects. The digitiser captures samples across several RRIs, forming a ‘frame’ of raw data that encodes the reflections from objects at different distances and angles.
Processing radar data: from raw echoes to clear images
The journey from raw radar data to a comprehensible image is a complex process involving several stages of signal processing. Initially, the raw, time-domain signal is transformed into its frequency-domain representation. This step is essential for identifying the unique ‘tones’ corresponding to different reflection distances in the captured radar signals. Subsequent processing stages focus on extracting useful information from the frequency-domain data, discarding irrelevant parts, and constructing visual representations that closely resemble the observed scene.
Visualisation and interpretation
Many modern radar systems are used for integration into automated systems, such as the monitoring and detection of drones, vehicles, and pedestrians. In these contexts, a radar provides a powerful sensor, though the value often lies not in the visual representation of data but in the functional application of the information gathered. Whilst visualisations serve as a bridge, translating the abstract radar data into plots for the users or developers—thereby building trust in the system or aiding in troubleshooting—many users look beyond these images. For instance, our Ubiquitous Radar (PLX-U16) offers a range of visualisations; yet the core demand from users is for succinct detection reports that can trigger immediate actions in other systems, such as directing a camera towards a detected object. The visualisation is not an end but a means to a broader capability: the practical, responsive action based on data interpretation.
Please accept cookies in order to enable video functionality.
This video provides an example radar output, where a single visualisation is shown alongside the feed from a co-located video camera.
Challenges and future directions
Even with big improvements in radar technology and how we handle radar data, there are still some tough challenges. One major issue is dealing with the huge amount of data that advanced radar systems create. This requires powerful computers and sophisticated software to process all that information quickly. Another challenge is in interpreting radar data in complex scenarios. For example, a radar can be used to detect drones, but it could be confused by birds, vehicles, and other moving objects. Here, AI (Artificial Intelligence) comes in handy. It can help figure out what exactly the radar has detected, for example telling the difference between a bird and a drone. By teaching AI to identify these differences, radar systems become much more useful and easier to work with.
The future of imaging radar technology promises further advancements in processing speed and resolution. With continued innovation, imaging radar systems will play an increasingly prominent role in shaping the technological landscape, driving advancements in autonomous systems, environmental monitoring, and beyond.
Conclusion & further reading
Through the lens of our advanced millimetre-wave radar demonstrator, the guide illuminates the intricate processes involved in transforming raw radar echoes into detailed images of the world around us. As imaging radar technology continues to evolve, mastering the art of radar data processing will remain a key skill for engineers and developers working at the forefront of technological innovation.
For a full exploration, the guide “A Programmer’s Introduction to Processing Imaging Radar Data”, by Matthew Roberts, provides an invaluable resource for understanding the nuances of imaging radar data processing.
Projects
View AllDownloads
View All Downloads- PLX-T60 Configurable mmWave Radar Module
- PLX-U16 Ubiquitous Radar
- Configurable IOT Framework
- MISPEC
- Cost Effective mmWave Radar Devices
- Antenna Design Services
- Drone Sensor Solutions for UAV & Counter-UAV Awareness
- mmWave Sense & Avoid Radar for UAVs
- Exceptional technology for marine operations
- Infrastructure Monitoring